Synthetic fuels: Are e-fuels an environmentally friendly alternative to gasoline?
Research into alternatives to gasoline and diesel is not limited to electric. For some time now, research has been carried out on so-called synthetic fuels, and car manufacturers – especially Porsche – are also getting more and more involved.
What are e-fuels?
E-fuels are a number of different electricity-based and synthetically produced fuels. They are artificially produced and a possible substitute for fossil fuels such as gasoline, diesel or kerosene. They can be used in conventional combustion engines or mixed with other fuels without further technical modifications and consist of hydrocarbon molecules.
Why are synthetic fuels being developed?
In order to achieve the climate goals set out in the Paris Agreement and various scenarios of the Intergovernmental Panel on Climate Change (IPCC), it is necessary to end the burning of fossil fuels in all sectors. In Germany, the aim is to reduce greenhouse gas emissions by 55% by 2030 and by 80 to 95% by 2050.
On July 18, Ursula von der Leyen wants to be re-elected EU Commission President and promises to allow e-fuels to be considered exceptions after the combustion engine phase-out. At least that's what she wants to campaign.
Direct electrification with renewable energies is not possible in all sectors. In such sectors, indirect electrification is therefore an option with the help of e-fuels.
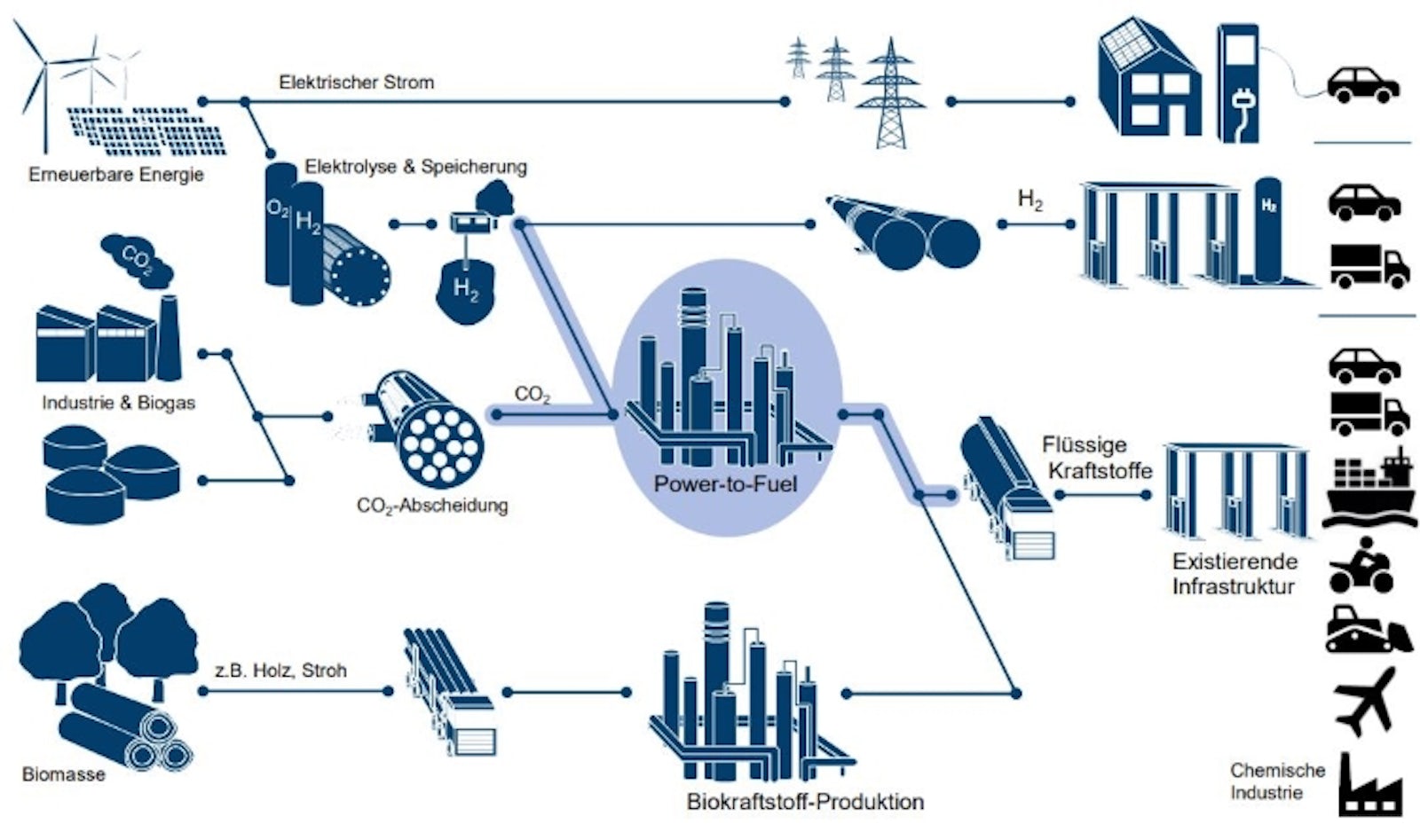
What synthetic fuels are there?
There is a whole range of synthetic fuels from hydrogen to a wide variety of e-fuels, which are usually categorized according to their area of application, e.g. e-gasoline, e-diesel or e-kerosene.
Furthermore, synthetic fuels can be used according to their CO2-Origin can be classified. In the discussion about sustainable e-fuels, reference is usually made to PtL fuels or StL fuels.
The following fuel types are differentiated as subforms of X-to-Liquid (XtL) technology:
- Gas-to-liquid (GtL fuels) usually made from natural gas
- Coal-to-liquid (CtL fuels)
- Biomass-to-liquid (BtL fuels) from straw, wood waste or energy crops
- Power-to-Liquid (PtL fuels) from green electricity using Direct Air Capture
- Sun-to-liquid (StL fuels) where solar energy is used for process heat
How are e-fuels produced?
The starting materials for the production of e-fuels are water (H2O) and Carbon dioxide (CO2). To put it simply, the water (H2O) by means of electrolysis in Hydrogen (H2) and oxygen (O2). By synthesis, the hydrogen obtained in this way is combined with the carbon molecules (C) of carbon dioxide to form hydrocarbon (CxHx).
Basically, there are two different production paths:
1. Fischer-Tropsch-Synthese (FT-Synthese)
Die FT-Synthese ist das gängigste Verfahren. Für dieses Verfahren wird zunächst ein Synthesegas aus Wasserstoff (H2), Kohlenmonoxid (CO), Kohlendioxid (CO2) und Wasserdampf hergestellt. Dabei werden hohe Konzentration an Kohlenmonoxid (CO) angestrebt. Aus diesem Synthesegas kann anschließend ein als E-Crude bezeichnetes Gemisch mit verschiedenen Kohlenwasserstoffketten entstehen. Das E-Crude kann von Raffinerien in spezifische Endprodukte als Ersatz für Benzin, Diesel oder Kerosin aufgetrennt werden.
2. Methanolsynthese
Methanol synthesis produces crude methanol, which can also serve as a crude oil substitute for other hydrocarbons. The most common is two-stage methanol synthesis, which also requires a syngas first. Direct methanol synthesis, which does not require the limiting factor of the reverse water gas shift reaction (RWGS) and thus without syngas, is rarer. Although further processing into end products such as diesel or kerosene is technically possible in methanol synthesis, this is not a standard today, unlike FT synthesis.
How efficient are e-fuels?
In order to compare the efficiency of different technologies, the efficiency is used. It indicates how much energy is supplied in the entire process and what percentage of it can still be converted into kinetic energy in the car at the end.
Process | Electric car (BEV) |
Hydrogen Vehicle (FCEV) |
Combustion engine (gasoline) |
Combustion engine (e-fuel) |
Renewable electricity | 100% | 100% | 100% | |
Transports / Line Losses / Processing Losses |
95% | 90% | 85% | 95% |
Electrolysis | 70% | 70% | ||
Fischer-Tropsch synthesis | 70% | |||
Hydrogen liquefaction | 80% | |||
Fuel cell | 60% | |||
Battery | 95% | 95% | ||
Electric motor | 90% | 90% | ||
Internal combustion engine | 30% | 30% | ||
System Efficiency | 81% | 26% | 26% | 14% |
Source: Federal Environment Agency based on WAGEMANN & AUSFELDER 2017
According to various studies, the efficiency of combustion engines powered by e-fuels is around 15% on average. On the one hand, this is due to the high losses caused by the long production chain and, on the other hand, it is limited by thermodynamics. There is little potential for increasing efficiency because most of the processes from the production of hydrogen to Fischer-Tropsch synthesis and the combustion engine have already been well researched.
By comparison, combustion engines powered by fossil fuels achieve an efficiency of around 25%. Fuel cell vehicles (FCEVs) that run directly on hydrogen reach 20 to 35%. Electric cars with an efficiency of around 75 to over 80% in some cases perform much better.
How environmentally friendly are e-fuels?
Theoretically, it is possible to make e-fuels as environmentally friendly as possible and CO2-neutral from renewable energies. To make this possible, all energy sources individually as well as the energy supplied must come from renewable sources. However, a better classification for the environmental badge is not to be expected with e-fuels.
Requirements for e-fuel production
The difficulties that exist in practice can be seen by looking at the individual requirements.
1. Electricity
Electricity from sustainable and renewable sources such as wind power, hydropower or solar is a basic prerequisite for the most environmentally friendly production of e-fuels.
The additional electricity demand that has to be met for e-fuel production is also relevant for the climate protection assessment. This argument is familiar from the discourse on electric cars and applies to e-fuels many times over due to their poorer efficiency. Thus, e-fuels are always in competition with alternatives, especially more efficient direct electrification.
In electricity systems with large shares of fossil and nuclear energy sources, it is likely that the additional electricity demand will be satisfied with quite high shares from these energy sources, resulting in higher greenhouse gas emissions or higher electricity generation shares from nuclear energy. The sole use of surplus electricity for e-fuel production would be uneconomical, especially because of the low utilization of the production plant, because "surplus electricity" only occurs in a few hours a year, in small quantities and spatially distributed. In addition, FT synthesis is a less dynamic process with few possibilities for responding to fluctuating renewable power generation capacities.
In the expansion of renewable energies to generate electricity, e-fuels are thus competing with other sectors. Globally, the climate protection impact of new renewable power generation capacities is higher if renewable electricity directly displaces fossil power plants in the domestic electricity system than if e-fuel is used. It follows that the export of e-fuels must not result in a time delay in the transformation of the electricity systems towards renewable energies in the respective country of production.
2. Hydrogen
There are two different processes for producing hydrogen. Potentially CO2only electrolysis, in which water is separated into the components hydrogen and oxygen, is -free. In addition to electricity generation, the production of hydrogen by electrolysis requires large quantities of water. Around 1.4 litres of water are produced directly for every litre of fuel produced in e-fuel plants. The water requirement increases indirectly to up to 70 liters of water per liter of fuel if the cooling of the individual processes and possibly also the cleaning of systems for solar power generation are included.
Die hohe Entnahme von Süßwasser aus umliegenden Gebieten für Lithium oder E-Fuels birgt ihrerseits fatale Folgen für die Umwelt, den Grundwasserspiegel und die Frischwasserversorgung in der jeweiligen Region. Viele der diskutierten Beststandorte für die Herstellung von E-Fuels sind günstig für die Stromerzeugung durch Solar oder Windkraft gelegen, gehören allerdings zu den trockensten Regionen der Welt. Die Wasserversorgung ist in diesen Regionen (z.B. MENA-Region, Südafrika, Australien, Teile Chinas, Südwesten der USA) bereits heute mangelhaft. Daher wird Wasserstoff für die E-Fuel-Produktion in der Regel nicht vor Ort produziert. Es fallen zusätzliche Transportwege, -kosten und -emissionen an.
Eine Entnahme von Salzwasser durch Anlagen in Küstennähe ist ebenfalls möglich. Nachteile ergeben sich dabei durch den abermals hohen Energieaufwand von Entsalzungsanlagen sowie die gleichen Umweltrisiken, die auch bei der Produktion von Lithium für Elektroautobatterien durch die Rückfuhr der mit Chemikalien und Salz angereicherten Sole entstehen.
Das weltweit am meisten verbreitete Verfahren zur Herstellung von Wasserstoff ist nicht die Elektrolyse, sondern Erdgasreforming. Dieses Verfahren trägt aufgrund seines fossilen Ursprunges nicht zur Reduktion der THG-Emissionen aus dem Verkehrssektor bei.
3. Kohlenstoffdioxid
Selten wird die Bereitstellung von Kohlenstoffdioxid (CO2) im öffentlichen Diskurs von E-Fuels thematisiert. Für ein Kilogramm synthetischen Kraftstoff werden etwa drei Kilogramm CO2 benötigt. Dabei zeigt sich, dass selbst bei einer Stromerzeugung aus über 95% erneuerbarer Energien, keinerlei Klimaschutzeffekt gegenüber fossilen Kraftstoffen erzielt werden kann, wenn ungeeignete Kohlendioxidquellen zum Einsatz kommen. Für den Bezug des Kohlendioxids kommen verschiedene Quellen in Betracht:
a. Direct Air Capture (DAC)
Ein klimaneutraler CO2-Kreislauf ergibt sich beispielsweise bei der direkten Entnahme aus der Atmosphäre bereitgestellt werden. Das sogenannte Direct Air Capture (DAC) Verfahren ist ein Prozess mit hohem Energieaufwand, denn die Konzentration von CO2 in the atmosphere is only 0.04%. The technology is still in the development phase, so it is associated with high costs and is only available in limited capacities. DAC plants also require a lot of space. This means that DAC is not sufficient as a source for the production of e-fuels on an industrial scale in the short and medium term.
b. Natural CO2-Sources
Another CO2-neutral source results in places where CO₂ naturally emitted into the atmosphere without human influence (e.g. geological sources). However, the potential of such sources is low, so that they do not result in significant amounts of carbon dioxide for e-fuel production.
c. Biogenic sources
The utilisation of biomass from wood or plants represents a further CO2source, which at least theoretically renewable. The supply of this biomass is limited and the demand as an energy source for space heating or as a building material is high. This results in a threat to the forests of the respective timber exporting countries or competition for the food supply in the cultivation of energy crops such as maize.
d. Industrial sources
The largest amounts of CO2 can be accessed via the Dual use of fossil energy provide. For example, the production of bioethanol and biogas produces high proportions of carbon dioxide in the exhaust gas streams, which can be separated with comparatively little energy. However, the wide spatial distribution of such plants would initially provide an infrastructure for transporting CO2 require. More concentrated point sources exist at steel and cement plants, which are very suitable sources of carbon dioxide for e-fuel production.
Nevertheless, CO2 from the dual use of fossil fuels non-climatic or CO2–neutral. Carbon capture has a negative impact on the efficiency of the primary industrial process. These additional emissions must be credited to e-fuel production. In addition, dual use could lead to a loss of incentives for direct electrification or transformation to renewable energies in the respective industrial sectors. These additional greenhouse gases, which result from the slower GHG reduction of industrial point sources, can also be attributed to e-fuels.
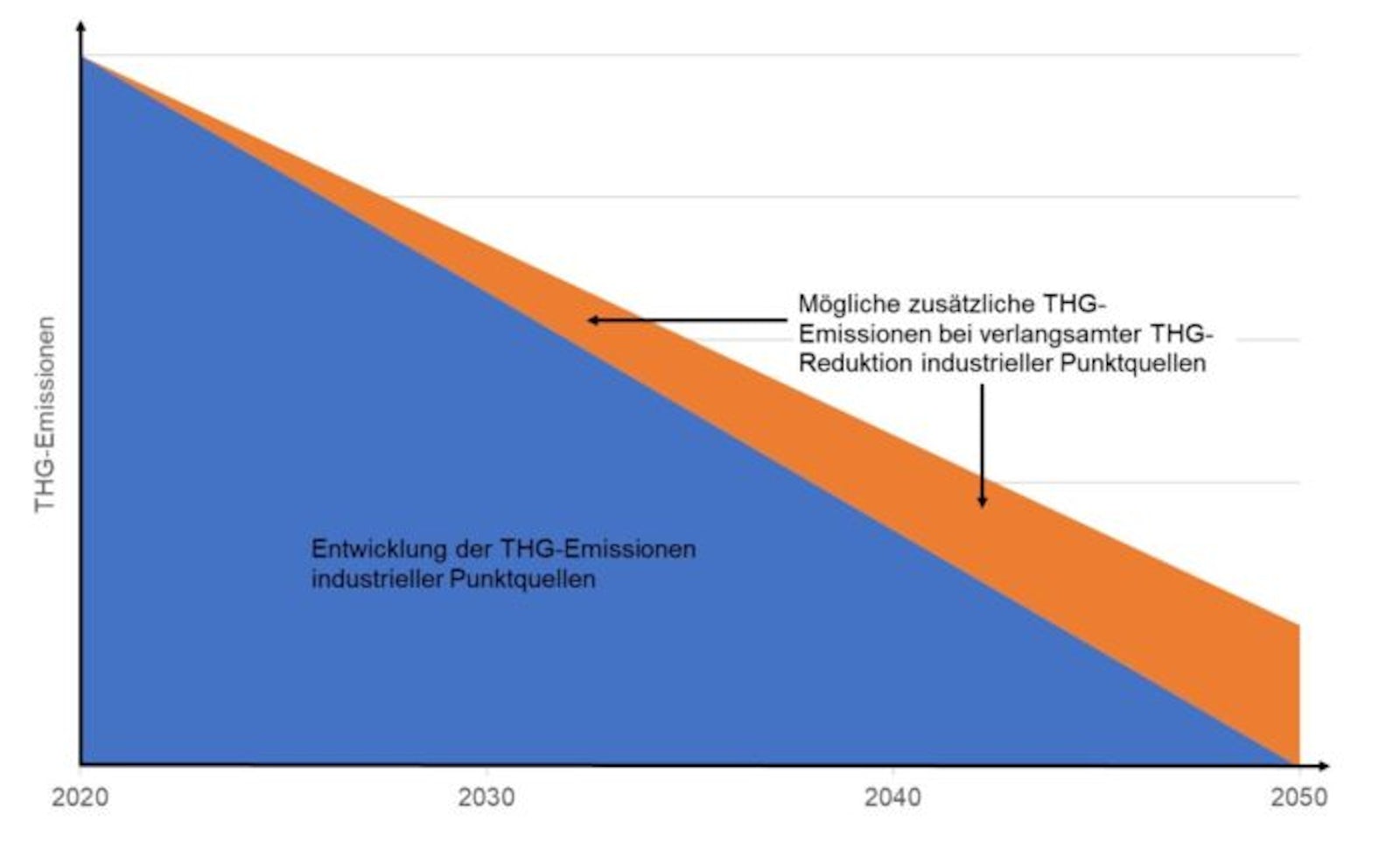
Furthermore, carbon dioxide extraction for the production of e-fuels as carbon capture and use (CCU) technology competes with carbon capture and storage (CCS) Projekten, bei denen das entnommene CO2 gespeichert (z.B. in den Boden gepresst) wird und dadurch nicht nur CO2-neutral, sondern CO2-negativ wirkt. Insofern darf die E-Fuel-Herstellung nicht Gelegenheiten zu Negativ-Emissionen mittels CCS verhindern, deren Nutzung laut Intergovernmental Panel on Climate Change (IPCC) essentiell für die Erreichung der Klimaschutzziele ist.
Im besten Fall, d.h. bei Nutzung erneuerbarer Energien im gesamten Herstellungsprozess und CO2 via Direct Air Capture, the provision of e-fuels still results in GHG emissions of 0.04 kg CO2eq (CO2equivalents) per kWh. They result, for example, from land use or the development of infrastructure. This is still around three times higher emissions than with direct electrification with renewable energies (0.014 kg CO2eq per kWh). If today's average electricity mix for production and the production of hydrogen via the common natural gas reforming is assumed, GHG emissions increase to 0.7 kg CO2Eq per kWh – ten times the amount produced by the provision of fossil fuels such as petrol, CNG and diesel (0.07 kg CO2eq per kWh).
Are e-fuels locally emission-free?
No. E-fuels can reduce CO2-neutral by reducing the CO2 elsewhere previously by Direct Air Capture (DAC) is taken from the atmosphere. Combustion in the engine in turn produces emissions. In addition to CO2 nitrogen oxides (NOx), carbon monoxide (CO) and ammonia (NH3). Compared to conventional gasoline engines, it can be seen that e-fuels emit just as much nitrogen oxides (NOx) as with the use of E10 and about a third compared to diesel. At the same time, the combustion of e-fuels produces three times as much carbon monoxide (CO) and twice as much ammonia (NH3), which can combine with other particles in the air to form particulate matter. E-fuels can therefore make no contribution to reducing local, harmful emissions in urban areas.
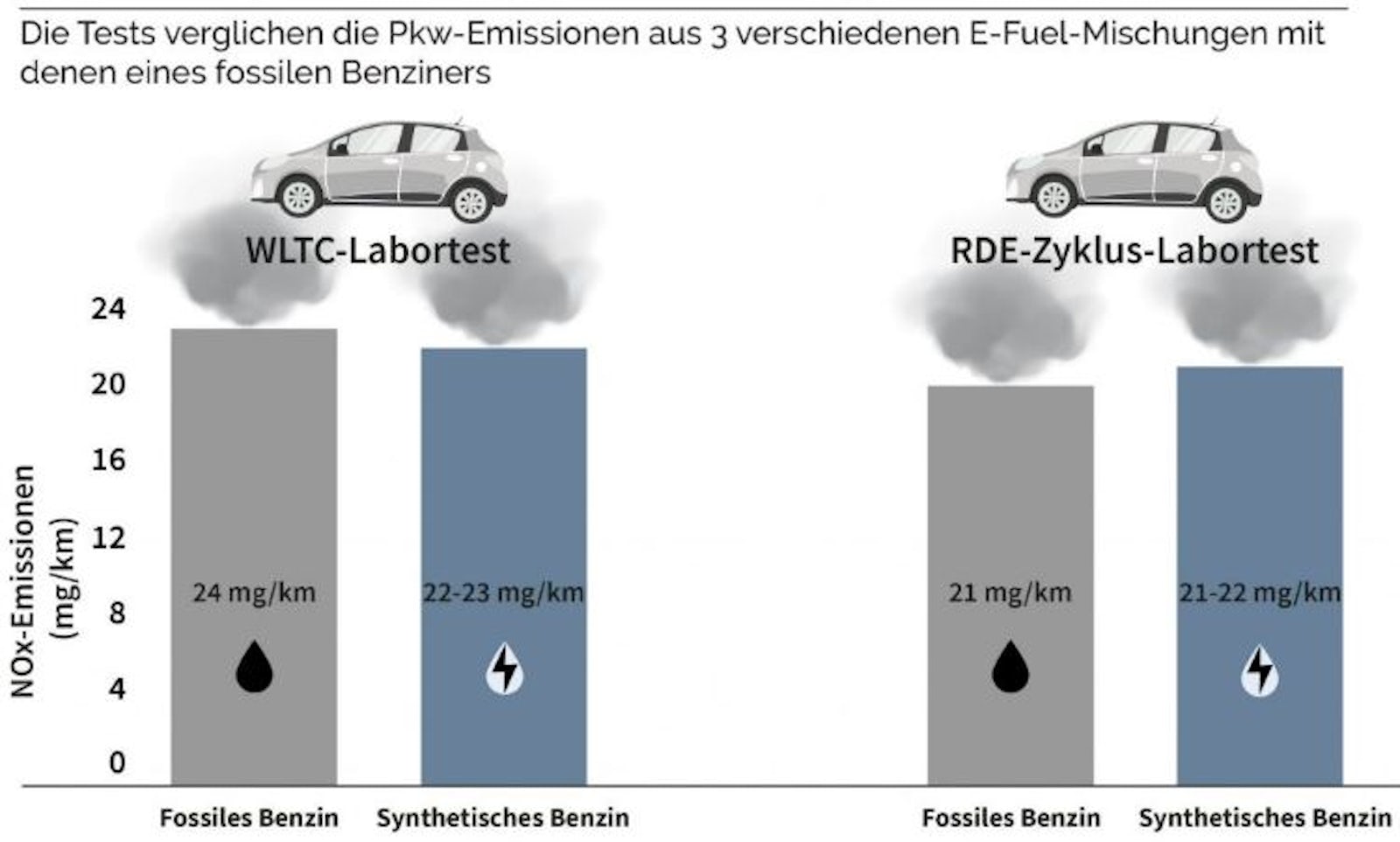
How much do e-fuels cost?
The high energy consumption makes e-fuels enormously expensive. The current production costs of the Haru Oni pilot plant in Chile currently amount to around €50 per litre of e-fuel.
By establishing e-fuels and associated processes, in particular Direct Air Capture (DAC), production costs could fall to around €2 per litre. This corresponds to four times the average wholesale price of fossil petrol (€0.50 per litre). In addition, there would be taxes and profit margins, so that the costs for end consumers would be significantly higher again.
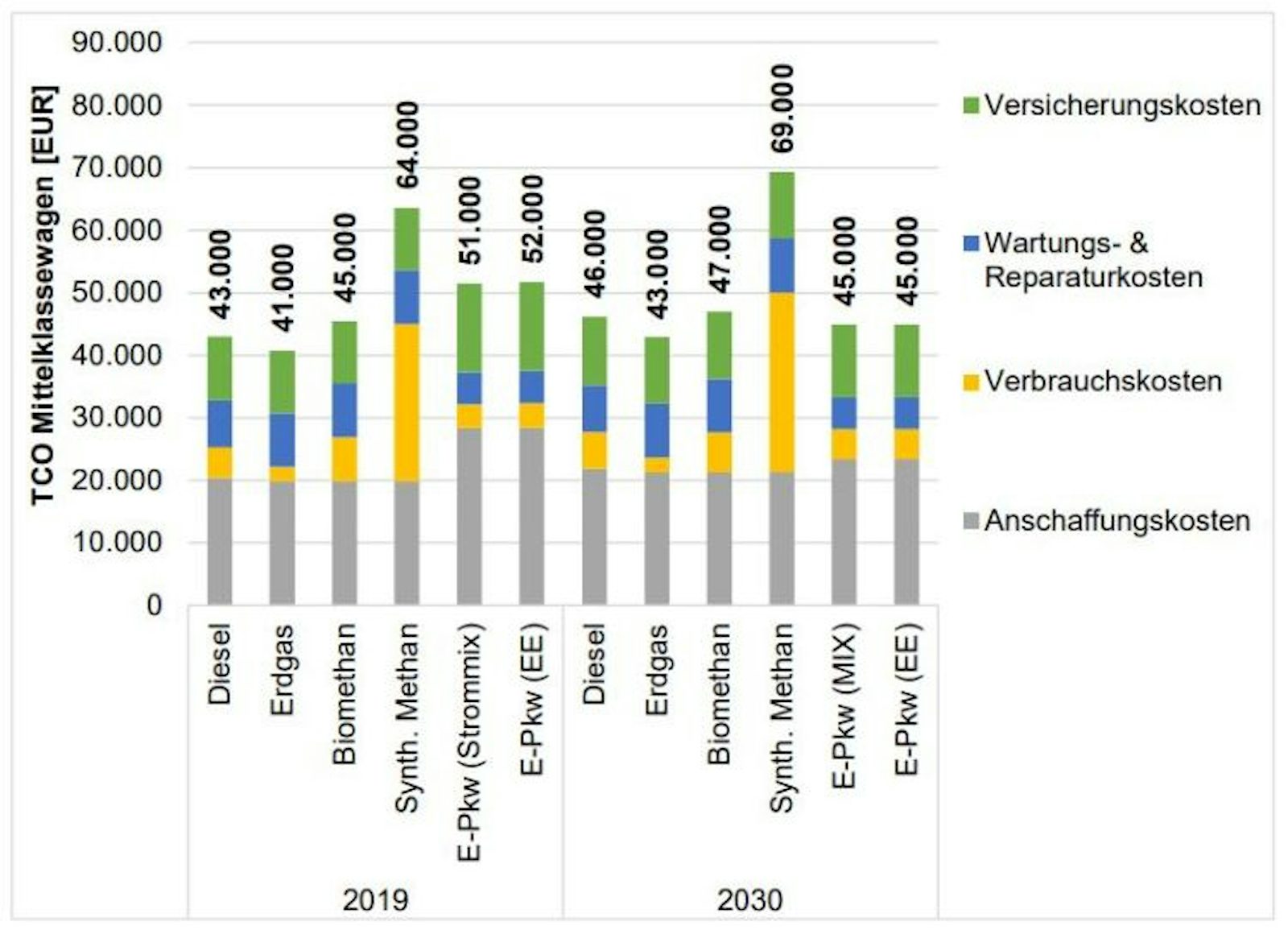
A comparison of all fuel types over the entire life cycle shows that cars with e-fuels are still the most expensive of all fuel types, even if e-fuel prices fall sharply. They would be more than 60% more expensive than the most cost-effective alternative (natural gas cars) and still 25% more expensive than electric cars. In a forecast up to 2030, the cost difference to e-cars will even increase to over 50% due to the falling production and acquisition costs for electric cars. This means that an e-fuel car would still be about 1.5 times more expensive than an electric car over its life cycle in 2030.
Are there any new cars that run on synthetic fuel?
E-fuels can be used in various forms as e-gasoline or e-diesel in conventional combustion engines without further modifications. Currently, there are no cars that run exclusively on synthetic fuel. There is currently no filling station in the EU that offers e-fuels.
The European Union's decision stipulates that from 2035 onwards, only cars with combustion engines may be newly registered if they can be operated exclusively with e-fuel and not with fossil fuels. Whether and how this restriction is technically feasible (i.e. that a car can check whether it is a fossil or synthetic fuel) remains unclear at present.
Where does the use of e-fuels make sense?
The low production capacities and the increasing demand for green fuels result in bottlenecks. The use of e-fuels contributes most to climate protection in sectors where unavoidable emissions are generated and direct electrification seems difficult or impossible: These are parts of the chemical industry for the production of plastics and, in the transport sector, especially aviation and shipping. There, electricity-based synthetic fuels offer the possibility of indirect electrification.
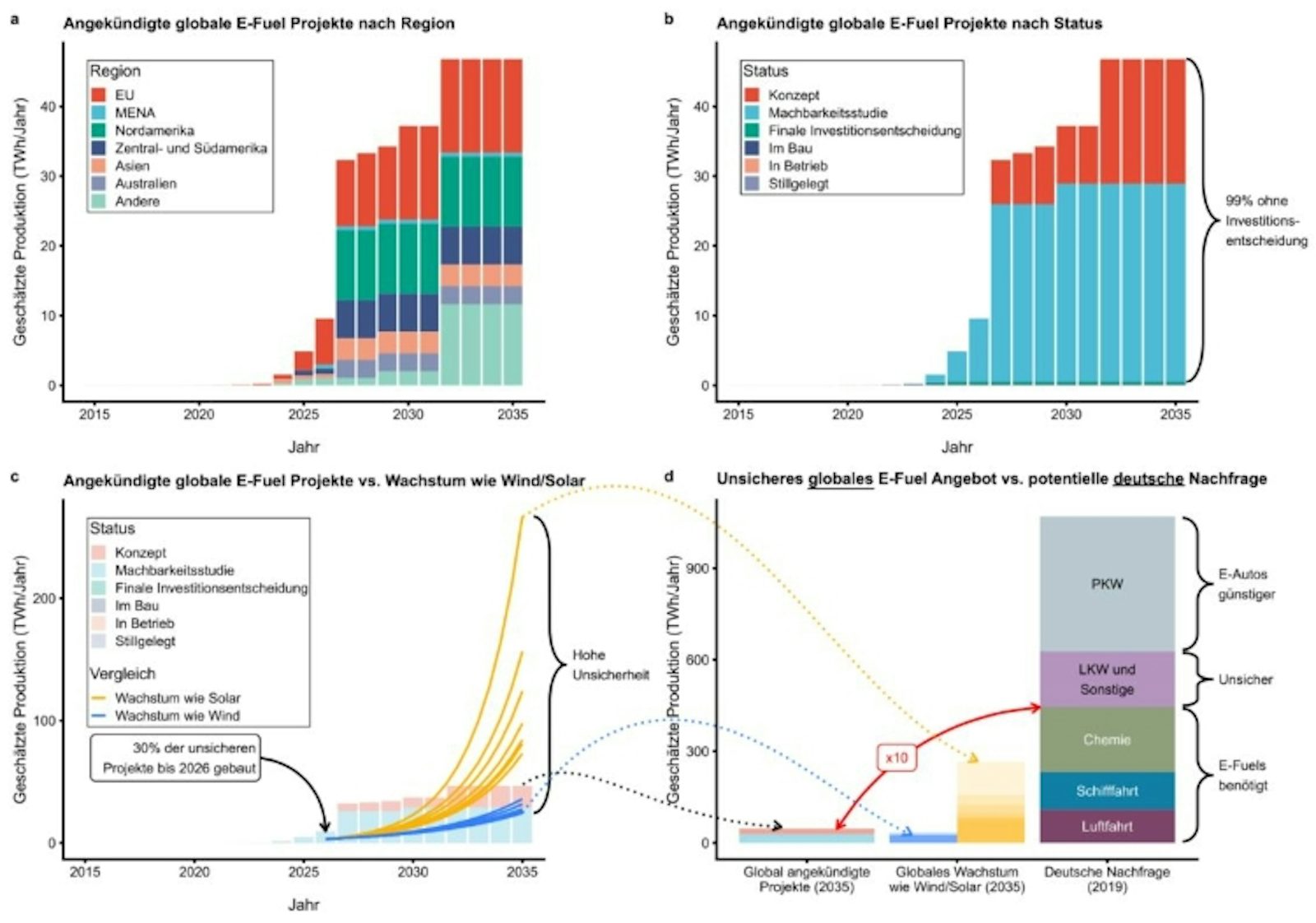
Bis 2035 werden derzeit weltweit etwa 60 neue E-Fuel-Projekte angekündigt. Der größte Teil davon befindet sich noch in Konzeption oder Machbarkeitsstudien. Nur für rund 1% dieser Projekte existiert bereits eine Investitionsentscheidung.
Wird optimistisch davon ausgegangen, dass alle angekündigten Projekte realisiert werden und sie ihre prognostizierten Produktionskapazitäten erreichen, so würde die globale E-Fuel-Produktion in 2035 nur etwa 10% der deutschen Nachfrage in unverzichtbaren E-Fuel-Anwendungen (Flugverkehr, Schifffahrt, Chemie) decken können.
In einem Szenario, in dem zusätzlich durch politische Anreize ein starkes technologisches Wachstum von 50% (vergleichbar mit dem Wachstum von Solar-PV) für E-Fuels angenommen wird, reichen die globalen Produktionskapazitäten 2035 noch immer bloß für die Hälfte der deutschen Nachfrage in unverzichtbaren Anwendungen.
It becomes clear that in the medium term no e-fuels will be available for car or truck traffic or that e-fuels used for cars and trucks are incompatible with the reduction of emissions in other sectors that cannot be directly electrified.